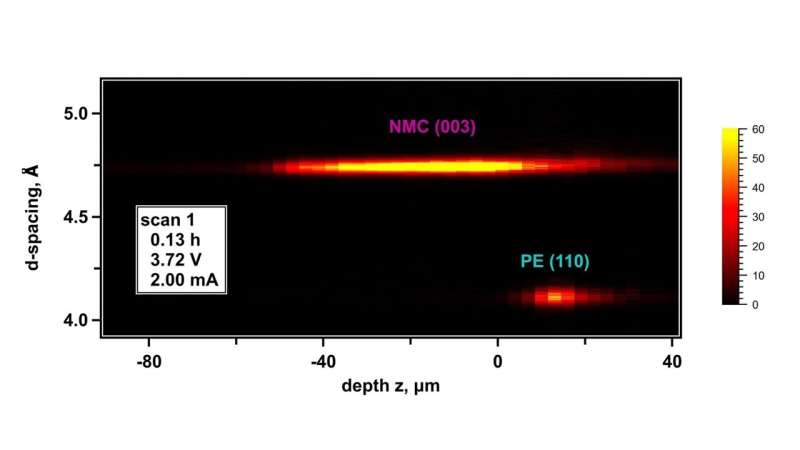
Researchers at the U.S. Department of Energy’s (DOE) Argonne National Laboratory have used a cutting-edge X-ray technique to view the movements of components inside an operating battery cell. The study is one of the first times that such movements have been directly observed in fine detail—at the scale of a millionth of a meter—while a battery charges and discharges.
“It’s really exciting to be able to visualize these movements,” said Daniel Abraham, a senior materials scientist in Argonne’s Chemical Sciences and Engineering (CSE) division and one of the study’s authors. “Other researchers have previously guessed that these movements happen, but they’ve not been able to show them in such fine detail.”
The challenge: ‘Moss’ growth inside a battery
A dizzying variety of battery types are vying to become one of the next generation of batteries. With applications in electric vehicles and energy storage for the electric grid, such batteries are an important part of the solution to reduce the effects of climate change.
At present, one of the more promising types of batteries is known as a lithium-metal battery. It substitutes a lithium metal anode (negative electrode) for the graphite anode now used in lithium-ion batteries. These anodes have ten times the energy storage capacity compared with graphite anodes but suffer from reduced performance after many charge-discharge cycles.
The Argonne researchers wanted to visualize the movements of battery components to better understand two challenges with lithium-metal batteries. First, these batteries tend to swell and shrink when charging and discharging. Second, there is a tendency for detrimental materials to build up on the lithium metal anode’s surface.
In their study, they viewed the movements of the anode, the cathode (positive electrode) and separator inside an operating battery cell. The separator is a porous layer in between the electrodes that prevents them from touching, which would otherwise lead to an electrical short.
When a lithium metal battery cell charges, lithium ions move from the cathode through the electrolyte to the anode. The anode expands as lithium atoms are deposited on its surface. During discharge, the anode contracts as lithium is stripped away. This back-and-forth process is normal.
“An ideal lithium battery would reversibly deposit and strip a uniform layer of lithium over thousands of charge-discharge cycles,” said Abraham. “Such a battery would store and deliver charge for many years.”
Lithium metal is highly reactive. When deposited on the anode, the metal quickly reacts with electrolyte nearby. These reactions form a layer of material over the lithium called the solid electrolyte interphase.
A thin interphase layer can protect a battery’s health. It separates the electrolyte and lithium metal so they cannot keep reacting. Such reactions could eventually consume all of the electrolyte—and the cell would stop working.
“A thin interphase is similar to an eggshell covering an egg,” said Abraham. “Just as an embryo in an egg can breathe the air that comes through the eggshell, lithium ions can still move through the interphase to continue the charge and discharge process.”
But the interphase material can become problematic if it gets too thick. As new lithium is deposited on the anode, more reactions can form more interphase layers. Different parts of the layers can grow into each other, forming little pores. These pores create additional surfaces and add to the volume of the interphase.
“Many of today’s electrolytes continue reacting with the lithium metal to form a thick mass of interphase material,” said Abraham. “This mass can hinder the movement of lithium ions through the anode and compromise the cell’s performance and cycle life. When we look at the thick interphase under a powerful electron microscope, it looks like a mat of moss on a forest floor. The moss is whiskers of lithium metal encrusted with the interphase material.”
“If you’re trying to design batteries for phones, computers and cars, you don’t want the irreversible expansion of the anodes to be too much,” said John Okasinski, an Argonne physicist in the X-ray Science division and one of the study’s authors. “That could cause problems with other components in the device.”
Using X-rays to see battery movements
At Argonne’s Advanced Photon Source, a DOE Office of Science user facility, the researchers used a cutting-edge technique called energy dispersive X-ray diffraction. This technique involved directing an X-ray beam into a lithium-ion battery cell as it was repeatedly charged and discharged. The cell was a small circular device known as a coin cell. It contained an anode made of lithium metal and a cathode made of a metal oxide material.
The researchers measured the changing patterns of the scattered X-ray beams. This information enabled them to map out and characterize changes in the atom arrangements in the cell’s materials. The team then used those arrangement changes to quantify the movements of the cell’s components.
“The movements we measured were caused by the anode’s expansion and contraction as lithium was deposited and stripped away,” said Abraham. “We found that the anode grew much larger than expected due to the buildup of the interphase material. We also saw that the expanding anode pushed against the cathode and separator, causing them to move as well.”
Valuable future applications for the advanced X-ray technique
The advanced X-ray technique in this study uses high-energy X-ray beams that penetrate the coin cell’s protective steel case and look deep inside a cell as it operates.
The researchers demonstrated that the technique can be used to quantify the amount of reversible lithium plating and stripping in an operating battery cell. It can also measure the amount of lithium metal permanently lost to the interphase layer—and the resulting growth of the interphase. These capabilities are extremely valuable because they can be used to screen for the most effective, next-generation electrolytes.
“Battery scientists can use the technique to quickly identify the best electrolytes,” said Abraham. “These would be the ones that form a thin interphase layer, prevent the mossy pileup of material and allow lithium metal to deposit and dissolve uniformly. One of our next research steps is to use the technique to evaluate how next-generation electrolytes can optimize lithium deposition and the interphase layer. We also plan to investigate how different anode surfaces—like copper—can alter and improve these processes.”
The X-ray technique has other valuable applications. It can identify if the expanding battery components are compressing the separator too much. This compression can cause the separator’s pores to close, slowing down or even stopping the movement of lithium ions between the electrodes. It can also be used to determine lithium concentration gradients in the cathode.
The findings are published in the Journal of Power Sources.
More information:
Ilya A. Shkrob et al, Visualizing electrode assembly movement and lithiation heterogeneity in lithium-metal batteries using operando energy dispersive X-ray diffraction, Journal of Power Sources (2022). DOI: 10.1016/j.jpowsour.2022.232273
Citation:
Scientists use X-ray beams to observe tiny movements, deep inside a lithium battery (2023, April 5)
retrieved 5 April 2023
from https://techxplore.com/news/2023-04-scientists-x-ray-tiny-movements-deep.html
This document is subject to copyright. Apart from any fair dealing for the purpose of private study or research, no
part may be reproduced without the written permission. The content is provided for information purposes only.
For all the latest Technology News Click Here
For the latest news and updates, follow us on Google News.