There’s a new measurement of muon magnetism. What it means isn’t clear
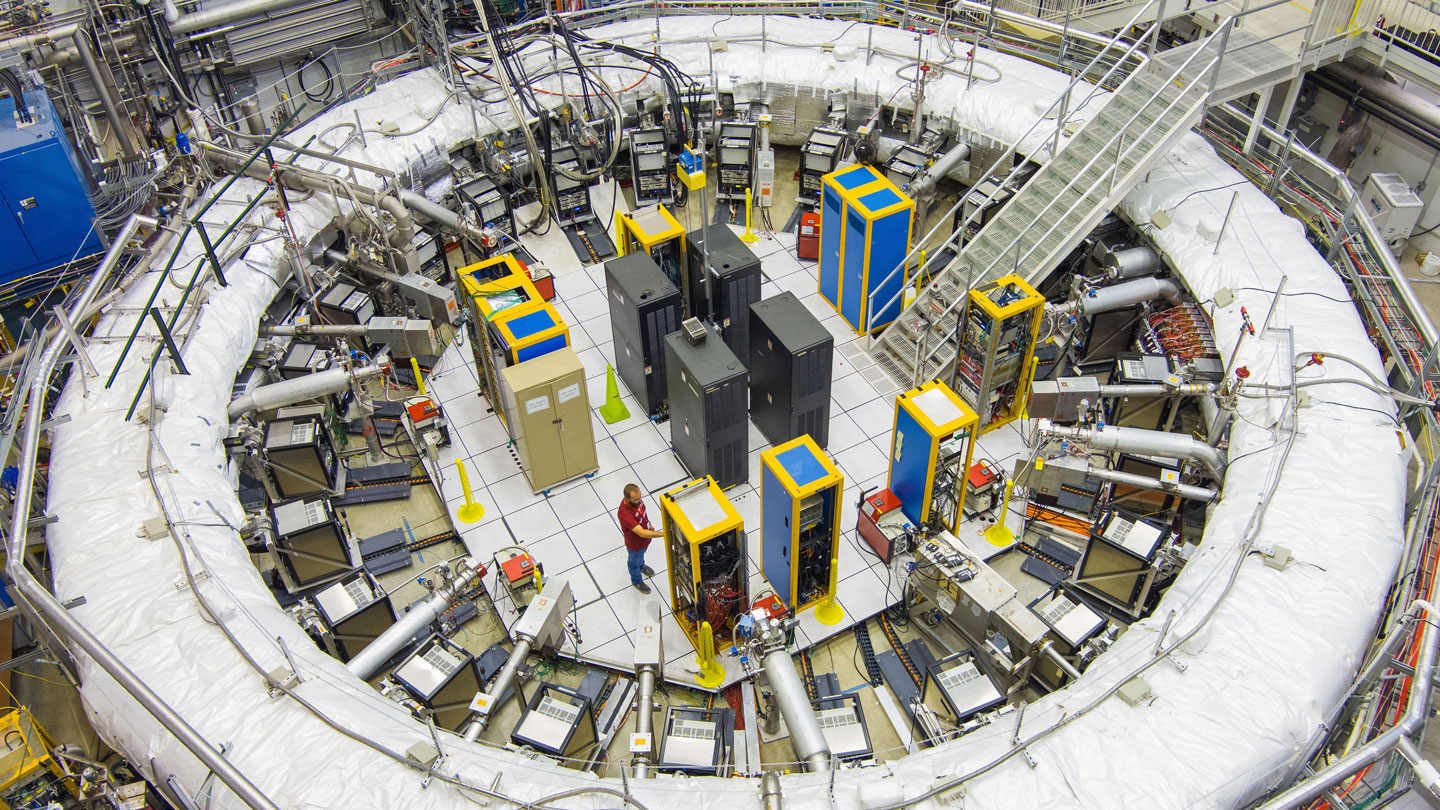
Muons might not behave as expected. But scientists can’t agree on what to expect.
By taking stock of how the subatomic particles wobble in a magnetic field, physicists have pinned down a property of the muon’s internal magnet to greater precision than ever before, researchers from the Muon g−2 experiment reported August 10 in a seminar hosted by Fermilab in Batavia, Ill.
Previous measurements of muons’ magnetism haven’t aligned with theoretical predictions. Those predictions come from one of the most important and carefully tested scientific theories ever developed, the standard model of particle physics, which describes subatomic particles and the forces that bind them.
Many physicists have hoped that the muon discrepancy might be hinting at a flaw in the stalwart theory that could lead to a better understanding of the universe. But several recent scientific surprises have muddled the theoretical prediction of the strength of the muon’s tiny magnet, making it harder to know if the measurement is pointing to new physics or an unresolved issue with the prediction.
Measurements of muon magnetism have long hinted at unknown particles
Muons are in the same particle family as electrons but are about 200 times as massive. These short-lived particles behave like miniature magnets, each with their own magnetic field. The strength of that magnet is adjusted by a strange effect of quantum physics. Empty space is filled with a constant flurry of particles that appear temporarily before flitting out of existence. Known as “virtual” particles, they have very real effects. These transient particles alter the strength of the muon’s magnet by an amount that can be calculated according to the standard model.
The precise value of this tweak — known as the anomalous magnetic moment, or “g−2” in physics equations — is what has befuddled physicists.
Tantalizingly, particles unknown to science could shift the value of g−2 that scientists measure. So previous hints of a disagreement with the standard model’s predictions have generated a hubbub among physicists.
“The muons’ behavior that we’re measuring is affected by all of the forces and particles in the universe,” says Muon g−2 researcher Brynn MacCoy, a physicist at the University of Washington in Seattle. “It’s basically giving us this direct window into how the universe works.”
The first indication of a mismatch between the prediction and measurements of g−2 came from an experiment at Brookhaven National Laboratory in Upton, N.Y., completed more than two decades ago (SN: 2/15/01). Then in 2021, the Muon g−2 experiment, based at Fermilab, reported its first results, confirming the discrepancy (SN: 4/7/21).
Now, Muon g−2 has doubled its precision in an updated magnetism measurement, the researchers reported in the Fermilab seminar and in a paper posted August 10 on the website of the Muon g−2 collaboration.
“To reach that level of precision is really unprecedented and really impressive,” says physicist Carlos Wagner of the University of Chicago, who was not involved with the experiment. “I am simply in awe.” The new measurement incorporates four times as much data as the previous one, among other improvements that beefed up the precision.
Scientists aim to compare that measured value to the standard model prediction. But determining what, exactly, the standard model predicts is complicated.
There is a tricky step to calculating the value of g−2
In 2020, after much careful consideration, a group of theoretical physicists called the Muon g−2 Theory Initiative came to a consensus prediction that they could compare with measurements. But since then, new, contradictory information has come out from other experiments and theoretical calculations, detailed in a statement posted August 9 on the Muon g−2 Theory Initiative’s website. That information has left the prediction uncertain.
“It’s not possible to make a comparison at this point and say whether the standard model agrees or disagrees with experiment,” says theoretical physicist Tom Blum of the University of Connecticut in Storrs.
The confusion hinges on a particularly challenging bit of the calculation of g−2. Known as the hadronic vacuum polarization, it refers to the adjustment resulting from a virtual photon emitted by the muon that splits into a quark and its antimatter partner, an antiquark. Quarks are a class of particle that make up bigger particles known as hadrons, including protons and neutrons. The quark and antiquark interact before annihilating back into a virtual photon.
Scientists have come up with two main ways of calculating this hadronic vacuum polarization term. The conventional way involves using certain experimental data as an input to the calculation. Those data come from experiments that measure how electrons and their antimatter particles, positrons, collide and produce hadrons. The results of such experiments are thought to be well understood.
But a recent experiment, CMD-3, at the VEPP-2000 particle collider in Novosibirsk, Russia, disagrees with those other experiments, researchers reported in February at arXiv.org. If this one outlier is correct, that would suggest that the hints of disagreement between muon measurements and the prediction might be weaker than thought.
A second way of estimating the thorny hadronic vacuum polarization term uses a method called lattice quantum chromodynamics. That technique involves mathematically splitting up spacetime into a grid in order to make calculations more tractable. Scientists have only recently managed to make such calculations precise enough for useful comparisons.
In 2021, a group nicknamed “BMW” published their calculation of the hadronic vacuum polarization contribution in Nature. That estimate pointed to a closer harmony between the prediction and measurement of g−2 and disagreed with the data-driven approach. But the technique demanded confirmation. Since then, other scientists have performed their own lattice calculations to check a portion of the BMW result. Those teams obtained similar results to BMW, boosting confidence in the lattice method.
The focus has now shifted away from scrutinizing the experimental measurement and is instead aimed at analyzing the disagreement among different theoretical techniques.
“The experiment has delivered,” says theoretical physicist Thomas Teubner of the University of Liverpool in England, a member of the Muon g−2 collaboration. Now, to figure out if muons are keeping with the standard model or cracking it, it’s up to the theoretical physicists, he says. “We have to get our house in order.”
For all the latest Technology News Click Here
For the latest news and updates, follow us on Google News.